The advancement of PFAS science has accelerated over the last 7–8 years compared to characterization and remediation of other contaminants such as trichloroethylene (TCE), which evolved over more than 3 decades. The industry has generated thousands of peer-reviewed papers, developed new technologies, and improved analytical methods. Many of the prominent environmental industry conferences have been dominated by PFAS subject matter.
PFAS are a family of thousands of synthetic fluorinated chemicals, 600 of which are routinely used in a wide variety of industrial, commercial, and occupational processes. They are used in aqueous film-forming foams for fire suppression as well as commercial products including waterproof clothing, stain-resistant carpeting, non-stick cookware, and some cosmetics, where there can be direct and prolonged exposure. PFAS are persistent in the environment and have been detected in human blood serum, drinking water supplies, and atmospheric fallout. They are the subject of more than 150 fish consumption advisories in our nation’s waterways.
More than 25 states have begun to publish criteria for soil, groundwater, and drinking water. EPA published a roadmap (opens in a new tab) with a proposed timeline for statutory, policy, and research actions. With such a strong industry focus, combined with an uncertain and changing regulatory structure, PFAS science is evolving rapidly in terms of analytical methodologies, investigation techniques, risk assessment concerns, and treatment strategies. Watch EA’s Environmental Insights webinar below to learn more. A brief summary follows the video.
Analytical Methods
Since 2009, the targeted PFAS list of analytical methods for solids and liquids has evolved with additions from EPA and other agencies. Many focus on measuring PFAS using liquid chromatography and tandem mass spectrometry. Most existing methodologies are being applied for drinking water, with fewer used for complex matrices or groundwater. EPA Draft Method 1633, published late in 2021, is the most complete and favorable, including 40 PFAS compounds. Despite this, there are still analytical challenges such as when performing field assessments or screening-level studies. Investigators will need to consider both EPA Methods 1633 and 8327 with considerations for cost, efficiency, and limits of quantification.
Other methods include:
- Total Oxidizable Precursor (TOP) Assay, which is a nontargeted, indirect method for measuring PFAS precursors for synthetic and environmental samples, both aqueous and solid.
- Total organofluorine (TOF) utilizing either combustion ion chromatography, graphite furnace atomic absorption spectrometry, or particle induced gamma-ray emission. Adsorbable organic flourine (AOF) is applied for aqueous samples using EPA Draft Method 1621, while extractable organic flourine (EOF) is used for solids. Most of the research projects that EA is currently working on are identifying AOF and EOF.
Other than cost, the main limitation with these analytical methods is the limited number of laboratories capable of providing these services and long turnaround times (TATs) associated with their sample backlog.
In addition to supporting characterization and treatment, robust data concerning specific PFAS behavior will support forensic analysis in identifying their source. The robust data resulting from the analytical methods described above are used not only to inform remediation strategies, but also to differentiate between sources using principal component analysis or machine-learning classification algorithms.
Remedial Investigation
Prior to 2020, advanced site characterization tools were generally used for recalcitrant legacy contaminant sites. PFAS has many unique characteristics—it does not degrade and migrates in groundwater long distances because contamination thresholds are so low. The industry is now using advanced tools, including high-resolution site characterization (HRSC) and environmental sequence stratigraphy (ESS), for PFAS sites to delineate faster and quicker during remedial investigation while improving conceptual site models. Applying these complex characterization techniques early on can save cost over the total project life cycle.
While site characterization focuses on scale (size of site, contaminant, pathways, and risk receptors), HRSC is a technique relying on the collection of data needed to account for site heterogeneity at refined scales. The goal is to provide more certainty for conceptual site models to support faster and more efficient clean-up.
With PFAS, it is critical to identify where the low permeability zones are located, where they can be retarded or sorbed, and the transmissive zones they flow through for targeting remediation scenarios. With an understanding of transport mechanisms in three dimensions, remedies can be tailored for varying locations—source, mid-plume, and toe of the plume—based on lithology. During the webinar, a case study is presented where HRSC and ESS were applied for a complex site.
Risk Assessment
The most important aspect of a risk assessment is toxicity values, which prevent significant challenges for PFAS. They have a wide range of effects by traveling through the blood to possibly impact nearly every organ and system in the body. This can include immune function; low birth weights; and liver, kidney, and thyroid disease. Effects differ depending on who is exposed. Half-lives of PFAS degradation differ greatly in humans versus animals as well as diverse genders and ages.
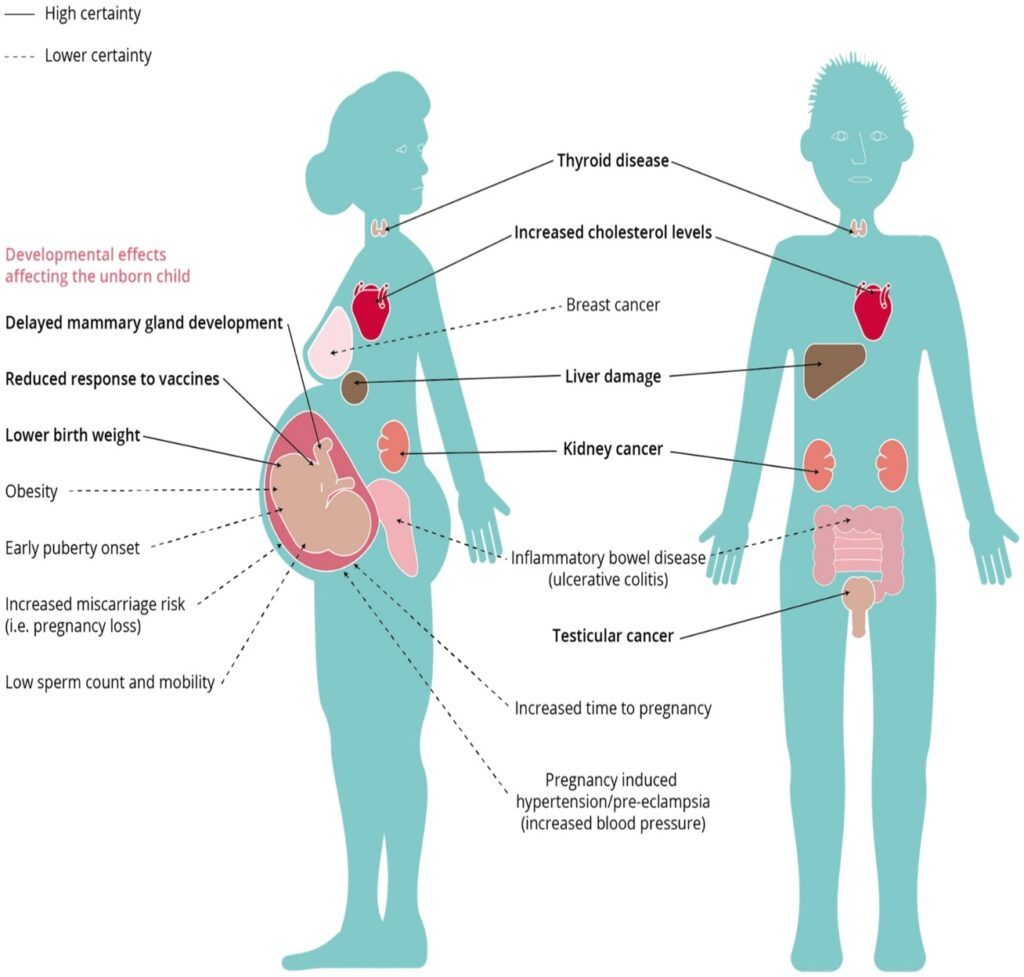
Source: Source Fenton Suzanne et all 2021
Health exposures are difficult to quantify. With thousands of PFAS, exposure occurs in a mixture and often throughout a lifetime due to its presence in food, consumer goods, and in the environment. Environmental justice communities are experiencing similar or, often times, worsening effects.
EPA released draft Maximum Contaminant Levels for PFOA, PFOS, PFBS, PFHxS, and HFPO-DA (“GenX”), which are based upon the level that can be reliably measured, not health endpoints. EPA has identified some of these compounds (e.g., PFOS and PFOA) as likely carcinogenic to humans; therefore, the Maximum Contaminant Level goal is zero. Supporting documentation for toxicity values currently differs from that shown on regional screening level tables. For instance, the non-cancer toxicity value for PFOA is 100 times lower than the value on the regional screening level table, and PFOS is 10 times lower. EPA is also in the process of evaluating additional PFAS and determining toxicity values. These changes will significantly impact previously completed risk assessments or site investigations that were completed using the previous toxicity or screening values.
Risk assessors also need to be looking at sources and sites perhaps not considered in the CERCLA process, including land-applied biosolids, wastewater discharges and spills, and fire training areas. Fish is another area of unique and complex exposure. Generally, the evaluation of fish ingestion in a human health risk assessment relies on modeling potential fish tissue concentrations from surface water uptake. This evaluation would then dictate whether the collection of fish samples is necessary. Researchers are finding the uptake from surface water to fish can be greater than 1,000 times, and also dependent on species and the specific PFAS mixture. Uptakes to agricultural crops, livestock, and wildlife are now beginning to be considered significant pathways.
Remediation Trends
The common method for PFAS treatment of drinking water is granular activated carbon as well as ion exchange resin. Foam fractionation shows promise as an emerging technology by taking advantage of the affinity of these compounds for the air-water interface. Using bubbles and foam, PFAS contamination can be skimmed off. The process benefits from its scalability and wide variety of applications. Other methodologies, such as reverse osmosis, membrane filtration, electrochemical oxidation, supercritical water oxidation, and ultraviolet-persulfate oxidation could be incorporated into larger treatment trains, though these technologies are currently not individually scalable for larger treatment.
For soil, there are several emerging strategies with the frontrunner being transport and disposal at permitted landfill facilities and thermal treatment. Thermal treatment, either by thermal desorption coupled with thermal oxidation or by incineration, is beneficial as it can completely and irreversibly destroy PFAS compounds. Uncertainty about off-gas emissions from thermal units is currently under intense investigation.
As the state of PFAS science continues to grow and evolve, new technologies are expected to emerge. Many are currently in research and development. A major focus in the future will be daily treatment of an estimated 34 billion gallons of daily wastewater discharges into our nation’s surface water.
According to the Environmental Business Journal, the projected cost of PFAS remediation over the next 20 years is in excess of $200 billion. As PFAS analytical detection sensitivity expands, and detection limits continue to lower, more contamination will be identified. As the industry continues its expansion of the science of PFAS, we will eventually catch up to regulatory criteria, factoring in treatment limitations, best management practices, limits of detection, and lessons learned.
For more information, contact:
Paul Caprio, P.G.
Vice President, Director, Chemicals and Contaminants of Emerging Concern
Contact Us
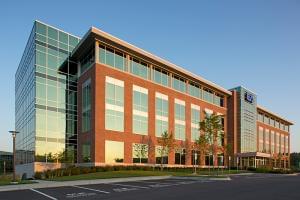